Dobsonian telescopes have long been a favorite among amateur astronomers for their simplicity, stability, and ability to gather light. But with a range of sizes of Dobsonians available, how do you choose the right one for you? In this article, we’ll delve into a comparison of three popular sizes: the 6″, 8″, and 10″ Dobsonians.
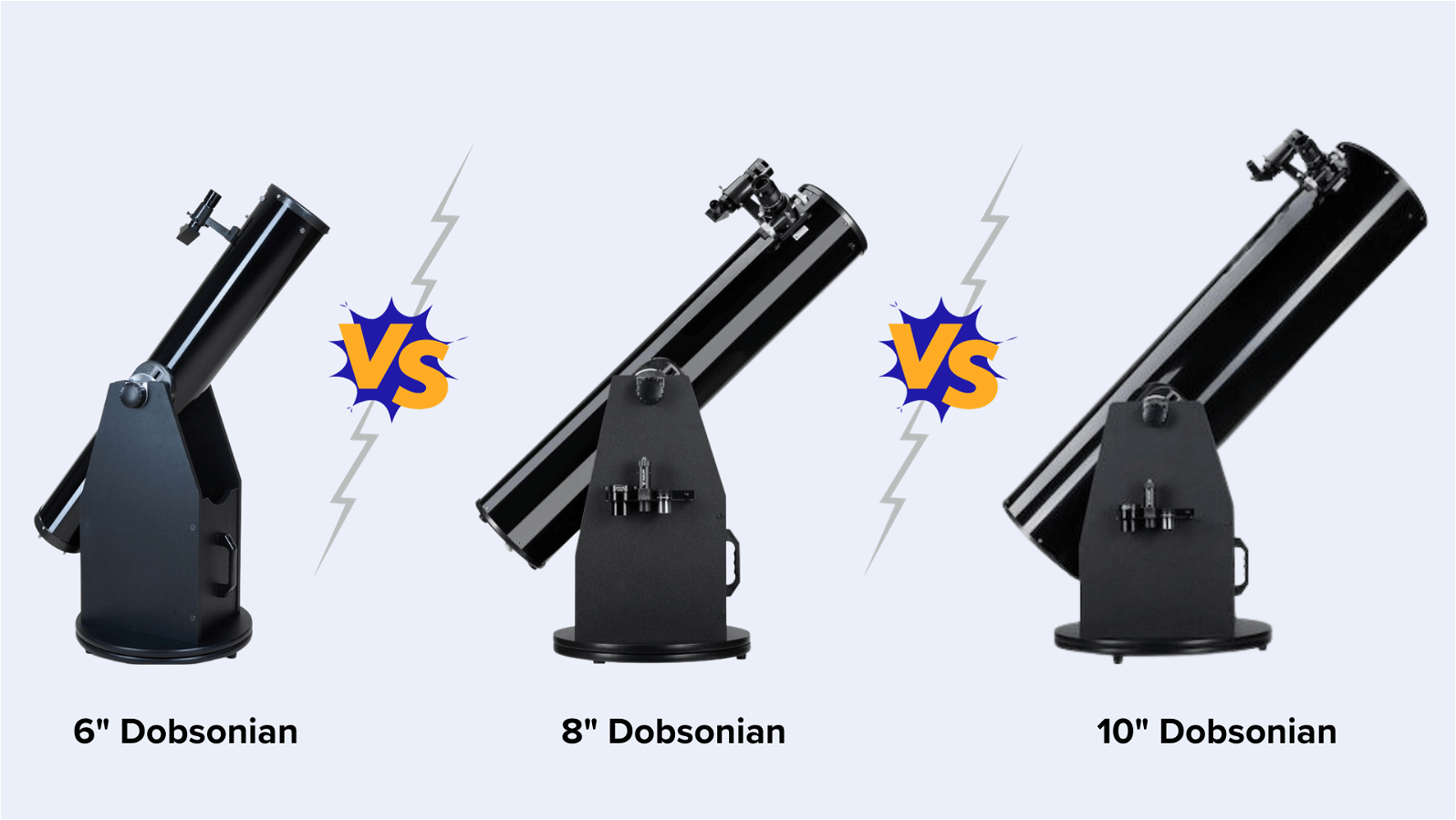
For many, the 10″ Dobsonian telescope emerges as the most cost-effective choice among these three traditional specs. Surprisingly, it also takes up roughly the same amount of space as its 6″ f/8 and 8″ f/6 counterparts, making it a practical option for those with limited storage. Yet, it provides a substantial step up in terms of light-gathering capability, allowing you to observe fainter objects and more detail on brighter ones.
The reality is simple: A 10-inch Dobsonian telescope offers substantially more light-gathering and resolving power than a 6” or 8” dobsonian telescope with negligible weight gain (a typical 10” is 50–60 lbs; a 6” freestanding (f/8) dob is 30–40). It also takes up negligible additional space and comes without a substantial price hike over either of the smaller apertures most of the time, too. If you can afford a 10” and can lift a 25-pound object, get the 10”. There is really no reason to go with an 8” Dobsonian over a 10” if price is not an obstacle, and really no particularly strong reason to buy a 6” freestanding (f/8) Dob whatsoever, given that they are not substantially cheaper or more compact than an 8” dob. Any performance improvements and conveniences of the smaller scopes, as we’ll get into in this article, are largely negligible, in part due to their similar physical frame. However, don’t discount the smaller 6″ tabletop Dobsonian telescope models.
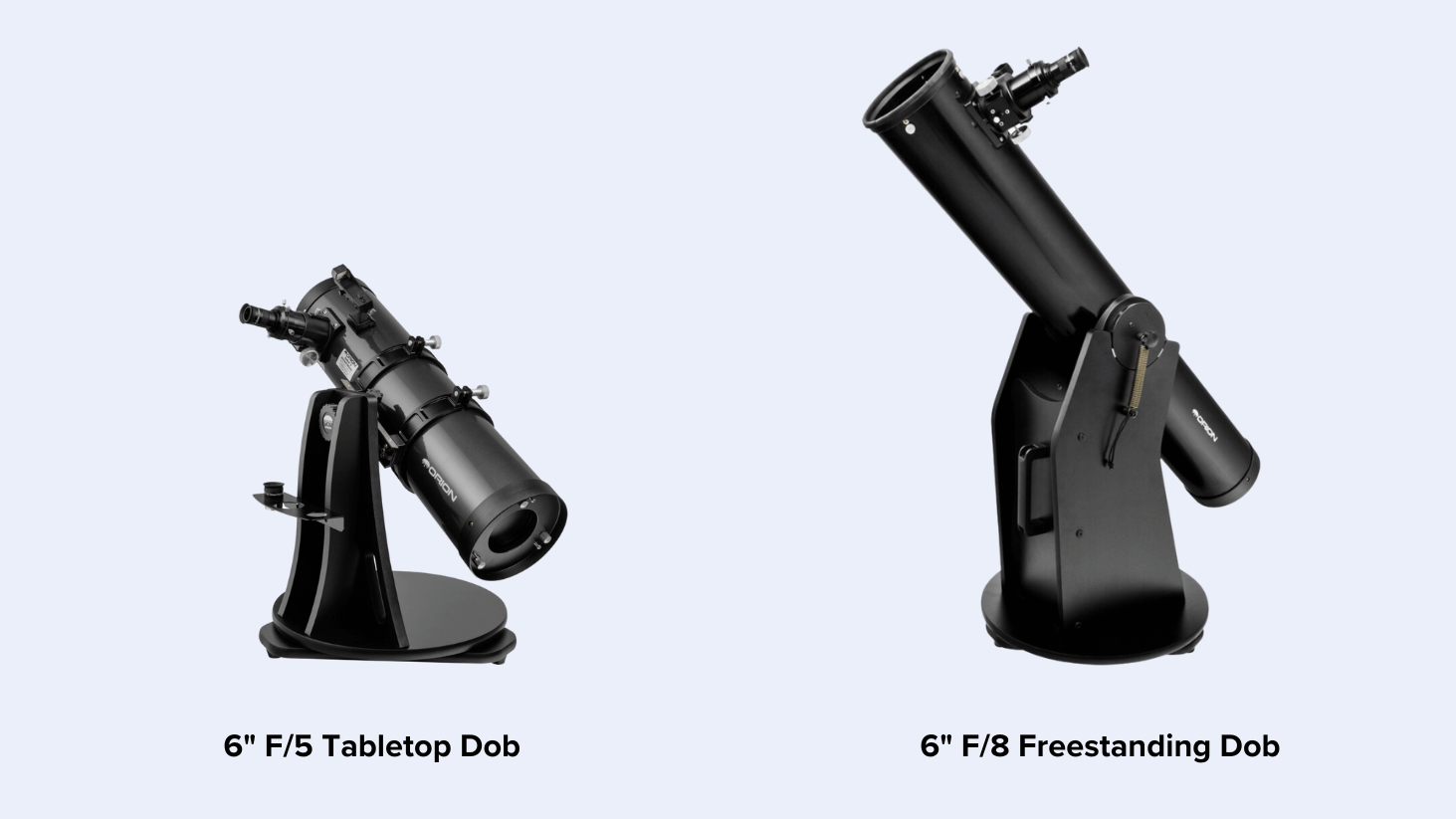
A 6″ f/5 tabletop Dobsonian is remarkably affordable and compact compared to the freestanding 6″ f/8 Dobsonians. It’s an excellent option for beginners or for those who prioritize portability, offering a delightful view of the night sky without the hefty price tag or the need for much space. A 6” f/5, or a smaller tabletop Dobsonian, is good to start out with if you can’t afford a 10” scope or as a “grab n’ go” complement to a 10″ scope.
If you are considering a Dobsonian telescope, regardless of size, it’s a good idea to check out our Best Telescopes and Best Dobsonians articles as well as our rankings. The Apertura AD10 is our top pick for a 6-10” freestanding Dobsonian as of the time of writing, but there are several models from a few different manufacturers at the 6”, 8”, 10”, and 12” sizes for you to check out.
While the allure of a 12″ model may be tempting, it’s essential to consider its intricacies. Truss tube designs, although providing greater portability for their size, can be complex and costly. On the other hand, more affordable solid-tubed 12″ models are noticeably bulky. For newcomers to the world of stargazing, this might not be the ideal first telescope, given its potential storage and transportation challenges.
Differences Light-Gathering Power Capabilities
In the world of amateur astronomy, the capacity of a telescope to gather light and resolve celestial objects is paramount. While telescope design, quality of optics, and various other factors play roles in determining a telescope’s performance, aperture size and focal ratio are often primary indicators of its potential.
Simply put, the light-gathering power of a telescope is directly related to the area of its primary mirror (or objective lens), which is proportional to the square of its aperture. A larger mirror collects more light, allowing the observer to see fainter objects.
The human eye dilates to about 7 mm in diameter under a dark sky. If you’re older, it’s more like 6mm, and people in their teens and twenties can have eyes that dilate to 8–9mm. A pair of 7×50 binoculars amplifies this aperture by about 50x, fed to each eyeball.
While 50mm binoculars would’ve impressed even Galileo, a modern 6” reflecting telescope gathers about 475 times more light than the average human eye. Ignoring slight variations in secondary mirror size, an 8” reflector dobsonian has about 78% more light-gathering power than a 6” reflector dob. Rather than list it all out, let’s have a look at the following table comparing each. These numbers are all approximations, and the limiting magnitude (the last 2 columns) varies heavily based on light pollution and, to a lesser extent, on magnification and/or the observer’s skill level.
Aperture of | Vs. 7mm Eyeball | Vs. 50mm Binoculars | Vs. 6” Telescope | Limiting Magnitude (Ideal) | Limiting Magnitude (Typical) |
Eye | 1x | 0.02x | 0.002x | 8x | 6.5x |
50mm Binoculars | 51 (x2) | 1x | 0.1x | 12x | 10.5x |
6” Dob | 475x | 9.5x | 1x | 14.5x | 13x |
8” Dob | 845x | 17x | 1.78x | 15x | 13.5x |
10” Dob | 1320x | 26x | 2.78x | 15.5x | 14x |
For simplicity, we are ignoring the factors of central obstruction and the ~92% reflectivity of modern mirror coatings, which most other estimates of light-gathering power do. The above estimates aren’t perfect, but they should give you some idea of what to expect.
Interestingly, regardless of what you use as a baseline reference, the jumps of an 8” over a 6” and a 10” over an 8” are pretty similar (78% vs. 56%), and this approximates to around half a magnitude or so of brightness in the real world.
Resolution Power of Different Dobs
Resolution is the telescope’s capability to distinguish two closely packed celestial objects or fine detail on an object.
Resolution scales directly with aperture, i.e., a 10″ telescope has twice the resolving power of a 5” telescope, assuming equal optical quality.
How exactly you measure resolution can vary; most astronomers use the Rayleigh criterion, which is an extremely conservative method of estimation but generally more applicable to all types of objects in the sky. Since we’re only comparing three sizes of telescope, we’ll give you the more optimistic Dawes limit as well:
Scope Aperture | Rayleigh | Dawes |
6” Dobsonian | 0.91 arcseconds | 0.76 arcseconds |
8” Dobsonian | 0.68 arcseconds | 0.57 arcseconds |
10” Dobsonian | 0.55 arcseconds | 0.46 arcseconds |
For comparison, the disk of Neptune is about 2 arcseconds, Saturn’s moon Titan is about 1 arcsecond across, and the disk of Jupiter spans about 40–50 arcseconds. There are 60 arc seconds in 1 arc minute, and the Moon or Sun are about 30 arc minutes, or a half-degree, across.
The Dawes limit is generally more applicable for high-contrast (i.e., easy) objects like equally bright double stars or surface features on Jupiter’s moons. The Rayleigh limit, on the other hand, applies more accurately to double stars of wildly different brightness, the subtle shades of Jupiter’s atmospheric storms, or in fine detail in dim, fuzzy planetary nebulae.
Theoretically, the increase in resolution linearly with respect to aperture applies boundlessly, but at larger sizes, you run into the brick wall that is the Earth’s atmosphere. More often than not, an “average” blurring of about 1-1.5 arc seconds is the norm for most locations. However, the atmosphere can appear to be completely still for brief moments, with disturbances falling back like a curtain for an eerily brief moment. It is during these moments that you can utilize the full or nearly full resolving power of your telescope to resolve close double stars or tiny lunar and planetary features. With larger apertures, however, these moments become short because the air is rarely stable enough to avoid distorting increasingly tiny details.
It’s also worth noting that columns of air tend to be about 8–12″ wide. This means that a 10” telescope falls right around the limit of resolving power on the bulk of nights, where the air is stable enough to allow for quality views but not exactly consistently steady either. A larger telescope can, in fact, provide a worse view, even with everything else being equal, simply because it has to peer through multiple columns of turbulent air. This is made worse by the fact that many larger telescopes have suboptimal optics, collimation, aren’t cooled down right, etc.
However, such a limitation only applies to viewing through the eyepiece. Planetary imagers can take videos and simply combine the moments of steady air into a wonderfully sharp picture that always benefits from the greater resolving power of a large aperture, even on a turbulent night. Likewise, on a sufficiently steady night, a large 16” or 20” Dobsonian can easily best even the best 10” in resolving fine detail.
The central obstruction in a Dobsonian or any other reflecting telescope, caused by the secondary mirror, can play a role in diminishing the contrast of the image. A larger central obstruction, often found in scopes with faster focal ratios like a 6″ f/5, can slightly degrade the image contrast compared to its 6″ f/8 counterpart. In theory, the 6″ f/5 and 6″ f/8 may have similar resolving powers. However, the 6″ f/8 might perform better when looking at high-resolution targets because it offers a little more contrast. The central obstruction of a 10” f/4.7 Dobsonian is proportionally smaller than that of either the 6” f/5 or f/8, since the secondary mirror needs to be large enough to illuminate a 1.25” or 2” diameter eyepiece in any telescope.
Portability
The ease of transporting and setting up a telescope is a key consideration. After all, no matter how impressive a telescope’s optics are, if it’s too cumbersome to move and deploy, it’s likely to spend more time in storage than under the stars. A 6” f/5 tabletop scope fits in a backpack, of course, but what about the larger scopes?
One of the first things you’d notice when you set three typical 6” f/8, 8” f/6, and 10” f/4.7 Dobsonians side by side is the uncanny resemblance in their physical forms. Their tubes are similar in length, as all hover around the 1200–1250mm focal length range optically, while the bases are similar in height, weight, and dimensions to cut on cost. The end effect is that the telescopes stand about equally tall. The uniformity in physical volume means that whether you’re packing a 6″ or a 10″ Dobsonian into your car for a night of stargazing, the space required remains essentially unchanged, and the same is true when these telescopes are not in use.
Of course, the difference does emerge when you consider tube girth and weight. As the aperture increases, so does the tube’s diameter and its associated weight. However, the progression from 6″ to 10″ isn’t as daunting as one might imagine. Even the 10″ tube, while being the heaviest of the trio, is manageable for most people at generally no more than 25 lbs (10 kg) or so.
The weight of the bases of most 6”, 8″, and 10” scopes also doesn’t scale very drastically; a 10” Dobsonian’s base is usually no more than 30 lbs, while that of a 6” hovers around 20 lbs.
Mostly commercial Dobsonian mounts are wildly mass-inefficient and lack even the most simple cutouts (the ground boards are also unnecessarily circular). The use of cheap, excessively heavy fiberboard or particle board for all of the fittings also increases weight. If you are really concerned about the weight of your Dobsonian’s base for some reason, anyone with basic carpentry skills and equipment can build a durable, lightweight, and more compact plywood rocker box for under $100 USD in a weekend.
Collimation Difficulty
Collimation, in simple terms, is the process of aligning a telescope’s optics for optimal performance. Many novices hear the term and immediately think of a complicated, time-consuming process. Add to that the presumption that a 10″ f/4.7 might be trickier to collimate than its smaller and slower siblings, and you can see where apprehensions arise about going big.
Here’s the reassuring truth: while different telescopes may have varying collimation sensitivities, the actual process is straightforward across the board.
With basic tools, such as a collimation cap or a good laser, and a little practice, the entire collimation procedure for any Dobsonian telescope can be done within minutes. So, even if you’re inclined towards the faster optics of the 10″, or a 6” f/5 tabletop Dob, don’t be deterred by fears of a collimation nightmare. With a bit of practice, it becomes second nature.
Differences in Cooldown Period
Cooldown time is the period required for a telescope to acclimate to the ambient temperature, ensuring that temperature differences within the telescope do not interfere with image quality.
It’s common to think that larger telescopes, with their heftier mirrors, would need significantly longer to cool down. While there’s a grain of truth here, the distinction isn’t as pronounced as some might believe. For one thing, it’s easy to add fans to larger telescopes, and for two, the difference between 6”, 8″, and 10” mirrors in terms of their overall thermal mass is low, particularly when they’re all made out of the same types of glass from the same places.
It is rare that you need to wait more than a half hour or so for the sharpest views with any Newtonian/Dobsonian telescope under 12”, even without the aid of fans and on a freezing night when the telescope is brought straight from indoors. Other optical designs can trap more warm air in the tube for longer periods, but not reflectors.
The easiest way to offset most cooldown times is simply to take your telescope out of your car or house early. By allowing your Dobsonian to begin acclimating while you attend to other setup details, such as collimation, you’ll often find that by the time you’re ready to observe, your telescope is too. The only caveat here is that waiting too long could allow your eyepieces to dew or frost up under damp conditions, so keep those warmed until they’re ready to use either inside or with dew heaters.
You can also start with objects that don’t demand the full sharpness of your telescope’s optics, such as nebulae or star clusters at low power, to give your optics extra time to acclimate.
Why are all of the 6”, 8″, and 10” Dobs the same height and focal length?
One might wonder why the 6″ (at least non-tabletop), 8″, and 10″ models all seem to hover around the 1200–1250mm focal length range. This convergence is not a coincidence; it is the result of a delicate balance between manufacturing limitations, practical usability, and economic factors.
Faster mirrors (those with a lower focal ratio) can, in many cases, be more challenging to manufacture than their slower counterparts. As the mirror’s curve becomes steeper, achieving a perfect parabolic shape becomes an increasingly delicate task. The 6″ f/8 and 8″ f/6 Dobsonians, both falling within our focal length range of interest, represent a balance; an f/8 mirror barely needs to deviate from a sphere, while an 8” is quite easy to manufacture.
Another factor is the telescope’s physical length. By maintaining a focal length in the 1200–1250mm range, these Dobsonians are tall enough for most observers to use comfortably without needing a table, yet still compact enough to fit in many cars.
The interesting economic twist is that the 6″, 8″, and 10″ Dobsonians often utilize the same hardware and accessories across product lines and often across brand names as well. Their similar sizes mean that packaging, shipping volumes, and even storage considerations don’t vary dramatically. Therefore, the major cost differentiation primarily stems from the primary mirror’s size and manufacturing complexity.
The 6″ f/8 and 8″ f/6 models, due to their comparable material costs and optical manufacturing tolerances, end up having a very marginal price difference. Essentially, you’re looking at two telescopes that, from a cost perspective, are nearly twins. This is why an 8” Dobsonian does not cost much more than a 6” f/8 (though the tabletop 6-inchers are considerably cheaper due to lower shipping costs). The typical 10″ f/4.7 to f/5 Dob, on the other hand, takes a step up in terms of cost, but not due to the hardware or the shipping—it’s the mirror. The 10″ primary mirror, being both larger and faster, poses greater manufacturing challenges, which are reflected in its price.
Why are 6” tabletop Dobsonians cheaper?
The 6” tabletop Dobsonians feature more difficult-to-manufacture f/5 primary mirrors and require a slightly larger secondary mirror than their 6″ f/8 counterparts, but no bigger than that of an 8” f/6 Dob. The key to why these scopes now (as of 2023) cost less is that the more compact design of a 6″ f/5 tabletop Dobsonian means that it’s generally cheaper to transport, both for the manufacturer and, ultimately, the consumer. This directly affects the final telescope’s price.
Before 2020, bulk container shipping costs from China to the USA and many other countries were much lower, so the marginal cost of shipping was not as major compared to the price of the goods themselves. Thus, tabletop 6” f/5 telescopes were often sold at more or less the same price as a 6” f/8. Today, there is a huge gulf in price between the sub-$400 or so tabletop Dobsonians and the larger 8-10” models. You may even notice this on our telescope rankings page.
What about larger Dobsonians?
The larger model above 10”, the common 12″ f/5 Dobsonian telescope, stands out as a more significant investment, and understanding its pricing requires a deeper dive into its physical structure and market dynamics.
The first consideration is the choice between a solid tube or a truss tube design. A 12″ solid-tube Dobsonian becomes quite hefty. The increased weight and volume don’t just impact portability; they also drive up shipping and handling costs. This weighty construction then balances against the truss tube designs, which, while more portable, come at a higher manufacturing cost due to their more intricate design, tighter tolerances, and the need for additional components. Both end up being a lot more expensive than would seem to make sense just scaling up from a 10”. Part of this is also due to lower demand, which forces prices to remain high to justify not simply canceling the product(s).
Larger telescopes, such as the 16″ models, face an intriguing market situation, which makes them far less common and far more expensive. The demand for these telescopes is, of course, lower than for their smaller counterparts, mainly due to the fact that they are expensive and bulky, though the latter is purely in the eye of the beholder and dictated by the telescope’s design.
However, one might ask, “Why doesn’t the industry respond by making them more affordable or compact?” It’s actually completely possible, but it becomes a circular economic conundrum. High production costs, combined with lower demand, make it less financially attractive for manufacturers to innovate in ways that could reduce costs. The resulting higher price tag of a new and innovative product can further deter potential buyers, maintaining the cycle of low demand. This is why you frequently see innovation coming out of home telescope makers such as compact 16-inchers, which cost and weigh less than a commercial 12” truss tube Dobsonian in a similarly or even less bulky form factor. The problems are purely demand-related rather than dictated by technology or cost.
While it’s obvious that there’s ample opportunity to innovate and make these larger telescopes cheaper or more compact, the financial incentives to do so are lacking. The combination of high production costs, niche market appeal, and the resulting pricing dynamics creates the status quo. Manufacturers are simply less motivated to invest in research and development for a segment of the market that already shows limited growth potential.